Original article
Published in spanish Científica Dental Vol. 16. Nº 3. 2019 www.cientificadental.es
Comparative densitometric analysis between a new bone graft material composed of calcium phosphate vs. bovine hydroxyapatite in alveolar ridge preservation. A pilot study
Introduction: In the present study, bone density from the new biomaterial composed by calcium phosphate and added silicon is compared with bovine hydroxyapatite by means of Hounsfield units in alveolar ridge preservation. Alveolar ridge preservation is a surgical technique proposed to reduce bone resorption caused by dental extraction, using a bone graft. This technique´s final goal is to facilitate implant insertion and rehabilitation.
Materials and methods: A study was carried out on 6 patients from the Faculty of Dentistry of the Complutense University of Madrid performing the technique of alveolar ridge preservation. Two groups were established, a test group in which the alveolar socket was filled with calcium phosphate and added silicon and a control group where the socket was filled with bovine hydroxyapatite. After 3 months, a cone-beam computed tomography was performed to evaluate the bone density achieved by both biomaterials.
Results: The average bone density achieved in the group treated with calcium phosphate and added silicon was 1100,40 ± 111,19 Hounsfield units whereas in the group treated with bovine hydroxyapatite the average bone density was 1029,46 ± 95,16 Hounsfield units.
Conclusions: Both biomaterials seem to present a similar behaviour in terms of densitometric results obtaining a density greater than 1000 Hounsfield units, having the calcium phosphate and added silicon the highest density.
The loss of alveolar bone can be triggered by different circumstances, such as trauma, an infectious process or as a consequence of periodontal disease. The most frequent cause of bone deficiency in the alveolar ridge is produced by the absence of mechanical function caused by the extraction or loss of a tooth1 .
Since the alveolar process depends on the presence of teeth, its loss leads to the unleashing of significant structural changes that are manifested in a vertical and horizontal reduction of the bone crest2, 3.
According to Seibert4 , alveolar ridge defects can be divided into three categories according to bone deficiency:
- Class 1: when bone deficiency predominates in the horizontal dimension.
- Class 2: when it predominates in the vertical dimension.
- Class 3: when it affects both vertical and horizontal dimensions.
Taking these into account, it has been observed that the horizontal bone component is the most affected after tooth loss, mainly affecting the vestibular cortex. Whereas, the crestal reduction in height is milder, and is also predominant in the vestibular cortex3 . Numerous studies have shown that most of the bone resorption, which occurs after tooth loss, occurs during the first 3 months of healing, and dimensional changes can be observed up to a year later2 .
Schropp et al,5 revealed that the bone crest loses 50% of its alveolar width during the first 12 months after tooth extraction, which represents a crestal reduction of 5 to 7 mm. Because of this, the ideal implant placement may be compromised2, 6.
Andrés-Veiga et al,7 observed that this amount of bone resorption can vary between one individual and another and even in the same individual at different times of life, due to the influence of a series of local and systemic factors. Post-extraction alveolar ridge preservation (PAP) is a surgical technique aimed at reducing the collapse of the alveolar ridge after tooth extraction with a biomaterial, to facilitate subsequent implant rehabilitation8-10.
Different types of biomaterials have been investigated for bone grafts in recent years, both in preclinical models and in clinical studies. However, none as yet has managed to stop resorption of the alveolar ridge completely after tooth extraction. The different studies carried out show that clinical, radiological and histological results vary according to the type of biomaterial used. Among such materials examined are autologous bone, allografts, xenografts, alloplastics and bone morphogenetic proteins10, 11.
Research lines, aimed at minimising or blocking bone resorption produced after tooth loss, have grown recently, due to the direct impact that this bone reduction has on the reliability of future dental implants12, 13. Adequate bone volume leads to a better chance of obtaining optimal aesthetics, thus reducing the need for additional grafts12.
A systematic review by Vignoletti et al,2 concluded that the PAP technique manages to significantly reduce crestal bone resorption in both width and height. The differences observed between the bone resorption of the groups treated with biomaterial and control groups, which were not treated, was 1.47 mm in height and 1.83 mm in width.
Despite this known reduction in bone resorption, there is still not enough scientific evidence to determine which alveolar filling biomaterial is superior in this technique2, 8, 14.
The aim of this study was to evaluate the bone density, measured in Hounsfield units (HU), achieved by a new biomaterial, composed of calcium phosphate with added silicon (CAPO-Si), and to compare it with bovine hydroxyapatite (HAB) in the PAP technique.
A retrospective cohort study was carried out on 6 patients with single or biradicular teeth susceptible to tooth extraction, and later rehabilitated with implants, from the Faculty of Dentistry at Madrid’s Complutense University. Two groups of 3 patients were formed: a test group having the socket filled with CAPO-Si after tooth extraction, and a control group in which it was filled with HAB.
In selecting the sample, the criteria were no patients having pathology or treatment that could compromise PAP (e.g. calcium disorders, treatment with immunosuppressants, bisphosphonates or corticosteroids; or radiotherapy or drugs that interfere with calcium metabolism). Furthermore, the integrity of the four corticals of the alveolus was necessary for their inclusion in the study.
After disinfection of the surgical field with povidone iodine (Betadine™, Meda Pharma SAU, Madrid, Spain), infiltration with articaine and epinephrine 40/0.01 mg/ mL (Ultracain™, Laboratorios Normon SA, Madrid, Spain) was performed. The tooth in question was then extracted, the integrity of all its cortices checked and the socket rigorously managed (Figure 1). Subsequently, using a full thickness flap, the alveolus was filled with the biomaterial in question (Figure 2) and a 25 x 30 mm size resorbable collagen membrane added (Osgide™, Curasan AG, Kleinostheim, Germany), see Figure 3. The flap was then closed using a 4-0 suture (Figure 4).
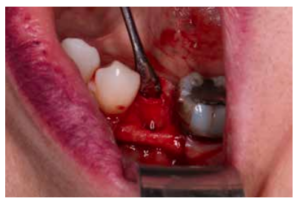
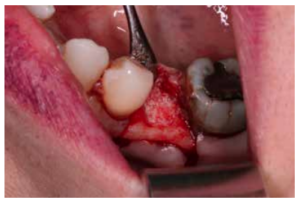
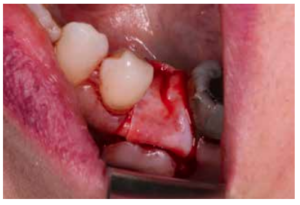
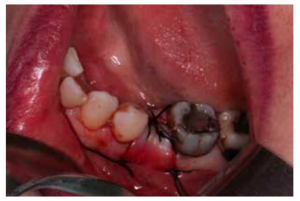
As postoperative measures, anti-inflammatory treatment (diclofenac sodium 100 mg every 12 hours for 4 days) and rinses with an antiseptic solution of 0.12% chlorhexidine (Chlorhexidine Lacer™, Lacer SA, Barcelona, Spain) were used. The patients returned 7 days later for suture removal and check-up appointments arranged during the first and second months using control periapical radiographs to evaluate correct tissue healing.
Three months after extraction, all patients underwent a cone beam computed tomography (CBCT) test (Newtom model 5G XL, Verona, Italy) to assess implant placement and the bone density achieved by the biomaterial (Figures 5 and 6). The densitometric measurements were performed with the NNT Viewer 7.2 program.

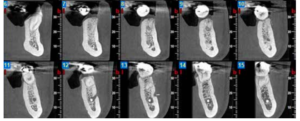
For statistical analysis, a Student’s t test was performed for independent samples after an evaluation of the homogeneity of variances. Data were presented as mean ± standard deviation and the level of statistical significance was established at p < 0.05.
In all cases, primary closure of the flap was achieved without any type of complication or exposure of the membrane being recorded during the follow-up period.
Three months after tooth extraction, the mean mineral density achieved in the CAPO-Si group was 1,100.40 ± 111.19 HU; while the HAB treated group recorded 1,029.46 ± 95.16 HU. The mean age of the test group was 53 years, and the mean age of the control group was 45 years. Table 1 shows the densities obtained by the study patients. Table 2 shows the mean and standard deviation values of the two groups and the Student’s t test results.
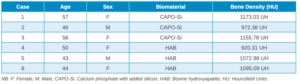
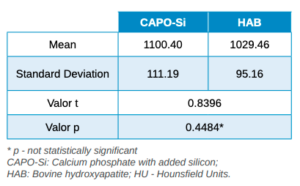
deviation values for the two groups and Student’s t test result.
Both groups demonstrated radiographically and clinically sufficient bone height for the mesial and distal ridges for implant placement. In addition, the width between the buccal and lingual cortices of the preserved alveoli was maintained, avoiding horizontal collapse of the alveolar ridge in both groups. None of the patients in the study showed dehiscence of the vestibular cortex.
Implant rehabilitation was possible in all cases 3 months after PAP; excellent primary stability was obtained without the need for additional bone grafting techniques.
In the present pilot study, the experimental biomaterial to perform the PAP treatment was a ceramic composed of CAPO-Si.
Silicon (Si) is an essential mineral for the proper growth and development of bones and cartilage. The justification for the incorporation of Si to the study biomaterial is due to the fact that it has been observed that those synthetic biomaterials based on calcium phosphate, which include levels of Si in their structures, demonstrate superior biological performance. This increase is attributed to the changes induced by Si in the properties of the material, since it stimulates osteoblast function and bone formation15. Furthermore, in different studies it has been observed that the incorporation of Si increases angiogenesis, promoting new bone formation16, 17.
The Patel et al study18 made a histomorphometric evaluation of the amount of newly formed bone by two compounds: one formed by hydroxyapatite and the other by hydroxyapatite with Si. The results revealed the compound with Si showed 15.5% more bone growth and 12.7% additional implant surface area covered by bone compared to the other without Si.
In this study, the combined use of membrane with the biomaterial is justified from a mechanical point of view, since it stabilises blood clotting, and acts as a support with a space maintenance effect to prevent epithelial growth. Also, it is biologically justified, since its composition provides an additional source of collagen, minerals and growth factors3 . The meta-analysis by Ávila-Ortiz et al (2014)11 on PAP concluded there are statistically significant differences in favour of using a membrane, as less vertical bone resorption was observed in the buccal and lingual cortices.
HU obtained from CBCT tests help evaluate bone density and quality and are used to evaluate the radiological density of materials, on a basis where air is < 1,000 HU, water is 0 HU, and the material with a higher density is > 3,000 HU. Most bone densities range from 100 and 1,900 HU19. Misch20 established a classification for evaluating bone quality using HU as follows:
- D1: bone with a dense cortex: > 1,250 HU.
- D2: dense to porous cortical bone and thick trabeculae: 850-1,250 HU.
- D3: thin porous cortex bone and fine trabeculae: 350-850 HU.
- D4: bone with fine trabeculae:150-350 HU.
- D5: bone with incomplete mineralisation: <150 HU.
The results obtained in this study show that the mean mineral density of the biomaterial in the CAPO-Si group was higher than that obtained in the HAB group: 1,100.40 HU and 1,029.46 HU, respectively. The biomaterial density for both groups corresponds to a type D2 bone, according to the Misch classification20. Due to the small sample size, no statistically significant differences were found in the density of the biomaterials.
These results are in line with similar studies in which PAP was performed. For example, Henao et al (2016)21 conducted a study on 37 alveoli in a test group using beta tricalcium phosphate, against a control group using a biphasic material of synthetic hydroxyapatite and tricalcium phosphate. CBCT tests performed at 3 months revealed a mean density of 1,052 HU for the group treated with beta tricalcium phosphate and 1,020 HU for the group treated with the biphasic material, with no statistically significant densitometric differences between the biomaterials.
In addition, the expected time for implant placement after PAP has been observed to vary notably between the different published studies. It could be argued that prolonged healing periods would help improve implant outcomes by allowing more time for bone tissue mineralisation in the socket. However, recent systematic reviews, such as that by Mardas et al in 201514 and De Risi et al in 20153 found no statistically significant differences in the survival and success rates obtained for implant studies with different healing periods after performing PAP. Thus, implant placement could be carried out after 3 or 4 months of healing, regardless of the bone graft materials used.
The sample size in this study was very small and, although our experimental group gave a higher densitometric mean than the control group, it was not a statistically significant difference. It would therefore be of interest to expand the sample in a future study, such as a clinical trial, and perform an additional histomorphometric analysis of both biomaterials to quantify the bone mineral density achieved and correlate it with the densitometric data.
Both study groups seem to have similar densitometric properties, with a density greater than 1,000 HU, and CAPO-Si giving the higher density.
Sanz M, Vignoletti F. Key aspects on the use of bone substitutes for bone regeneration of edentulous ridges. Dent Mater 2015; 31 (6): 640-7.
Vignoletti F, Matesanz P, Rodrigo D, Figuero E, Martin C, Sanz M. Surgical protocols for ridge preservation after tooth extraction. A systematic review. Clin Oral Implants Res 2012; 23 Supl 5: 22-38.
De Risi V, Clementini M, Vittorini G, Mannocci A, De Sanctis M. Alveolar ridge preservation techniques: a systematic review and meta-analysis of histological and histomorphometrical data. Clin Oral Implants Res 2015; 26 (1): 50-68.
Seibert JS. Reconstruction of deformed, partially edentulous ridges, using full thickness onlay grafts. Part II. Prosthetic/ periodontal interrelationships. Compend Contin Educ Dent 1983; 4 (6): 549-62.
Schropp L, Wenzel A, Kostopoulos L, Karring T. Bone healing and soft tissue contour changes following single-tooth extraction: a clinical and radiographic 12-month prospective study. Int J Periodontics Restorative Dent 2003; 23 (4): 313-23.
Jung RE, Ioannidis A, Hämmerle CHF, Thoma DS. Alveolar ridge preservation in the esthetic zone. Periodontol 2000 2018; 77 (1): 165-75.
Andrés-Veiga M, Barona-Dorado C, Martínez-González MJ, López-Quiles J, Martínez-González JM. Influence of the patient’s sex, type of dental prosthesis and antagonist on residual bone resorption at the level of the premaxila. Med Oral Pat Oral Cir Buc 2012; 1 (17): 178-82.
Horowitz R, Holtzclaw D, Rosen PS. A review on alveolar ridge preservation following tooth extraction. J Evid Based Dent Pract 2012; 12 Supl 1: 149-60.
Mardas N, Chadha V, Donos N. Alveolar ridge preservation with guided bone regeneration and a synthetic bone substitute or a bovine-derived xenograft: a randomized, controlled clinical trial. Clin Oral Implants Res 2010; 21 (7): 688-98.
Horváth A, Mardas N, Mezzomo LA, Needleman IG, Donos N. Alveolar ridge preservation. A systematic review. Clin Oral Investig 2013; 17 (2): 341-63.
Ávila-Ortiz G, Elangovan S, Kramer KW, Blanchette D, Dawson DV. Effect of alveolar ridge preservation after tooth extraction: a systematic review and meta-analysis. J Dent Res 2014; 93 (10): 950-8.
Iocca O, Farcomeni A, Pardiñas-López S, Talib HS. Alveolar ridge preservation after tooth extraction: a Bayesian Network meta-analysis of grafting materials efficacy on prevention of bone height and width reduction. J Clin Periodontol 2017; 44 (1): 104-14.
Tan WL, Wong TL, Wong MC, Lang NP. A systematic review of post-extractional alveolar hard and soft tissue dimensional changes in humans. Clin Oral Implants Res 2012; 23 Supl 5: 1-21.
Mardas N, Trullenque-Eriksson A, MacBeth N, Petrie A, Donos N. Does ridge preservation following tooth extraction improve implant treatment outcomes: a systematic review: Group 4: Therapeutic concepts & methods. Clin Oral Implants Res 2015; 26 Supl 11: 180-201.
Pietak AM, Reid JW, Stott MJ, Sayer M. Silicon substitution in the calcium phosphate bioceramics. Biomaterials 2007; 28 (28): 4023-32.
Alt V, Kögelmaier DV, Lips KS, Witt V, Pacholke S, Heiss C y cols. Assessment of angiogenesis in osseointegration of a silica-collagen biomaterial using 3D-nano-CT. Acta Biomater 2011; 7 (10): 3773- 9.
Zhai W, Lu H, Chen L, Lin X, Huang Y, Dai K y cols. Silicate bioceramics induce angiogenesis during bone regeneration. Acta Biomater 2012; 8 (1): 341-9.
Patel N, Best SM, Bonfield W, Gibson IR, Hing KA, Damien E y cols. A comparative study on the in vivo behavior of hydroxyapatite and silicon substituted hydroxyapatite granules. J Mater Sci Mater Med 2002; 13 (12): 1199-206.
Jeong KI, Kim SG, Oh JS, Jeong MA. Consideration of various bone quality evaluation methods. Implant Dent 2013; 22 (1): 55-9.
Misch CE. Contemporary implant dentistry. 2º Ed. St Louis: Mosby; 1999.
Henao SL, Morales LM, Valencia C, Arce S, Jaramillo A, Cruz C y cols. Determination of changes in height and bone density after process preservation with synthetic bone substitute. Rev Estomatol 2016; 24 (1): 11-7.
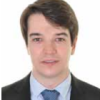
Cadenas Vacas, Guillermo
Graduate in Dentistry. Studying Master’s of Oral Surgery and Implantology. Complutense University Madrid (UCM).
Sanz Alonso, Javier
Collaborating Professor in the Master’s of Oral Surgery and Implantology. Faculty of Dentistry. UCM.
Martínez Rodríguez, Natalia
Professor in the Master’s in Surgery and Implantology, Hospital Virgen de La Paloma, Madrid.
Fernández Cáliz, Fernando
Professor in the Master’s in Surgery and Implantology, Hospital Virgen de La Paloma, Madrid.
Martínez-González, José María
Full Professor of Maxillofacial Surgery, Faculty of Dentistry, UCM.
Indexed in: – IME – IBECS – LATINDEX – GOOGLE SCHOLAR